Table of Contents
4-2 Glaciation
Read Chapter 16 in Physical Geology.
We are lucky to live during a time of glaciation because glaciers and their erosional effects are both spectacular and fascinating. They also hold a vast storehouse of information about the atmosphere and climate over the past several hundred thousand years. Even a few million years ago, glaciers were restricted to Antarctica and Greenland. Glacial ice did not exist anywhere on the planet 50 million years ago, nor for 200 million years prior to that. In addition, whereas many glaciations occurred in the distant past (Figure 16.1.1), the cumulative duration of glaciation on Earth represents considerably less than 10% of its history.
Section 16.1 covers the history of glaciations, and Figure 16.1.2 summarizes the climate record of the past 65 Ma. As already noted, it was warm during almost all of the Mesozoic, and well into the Cenozoic, but the temperature started to change around 50 Ma as the collision between India and Asia created the massive Himalayan Range and Tibetan Plateau. The enhanced rate of erosion produced by this uplift increased the rate of chemical weathering of silicate minerals, and because that process consumes atmospheric carbon dioxide, the global temperature started to drop. A number of other tectonic processes, including the formation of other mountain ranges, contributed to a drop in global temperature of more than 12˚ C over the past 50 million years, and to progressively more glaciation, first in Antarctica, then Greenland, and finally over much of North America and Eurasia.
As shown on Figure 16.1.4, the Earth’s average temperature has fluctuated dramatically over the past few million years, swinging between glacial periods with mean global annual temperatures around 5 to 6˚C, and interglacials—such as the present one—with mean annual temperatures around 12˚C. Those relatively short-term fluctuations are related to small changes in the Earth’s tilt and the shape of its orbit around the Sun, known as Milankovitch cycles.
Completing Exercise 16.1 will help you understand this cyclicity. In addition to answering the question asked, describe the nature of the temperature changes that preceded each of the past several glacial periods.
Section 16.2 in the textbook includes a discussion of the types of glaciers, and the mechanisms for glacier motion. Glaciers are of two types: alpine glaciers, which are present in mountainous regions, and continental glaciers, which cover entire continents. The only two real continental glaciers are those on Antarctica and Greenland. An ice cap glacier is similar to a continental glacier but covers less than 50,000 km2. They are present in Iceland, Svalbard, and the Canadian Arctic islands. An ice field is also a large area of ice, but one that is constrained by mountains. There are numerous ice fields in the Rockies and Coast Range of western Canada (Figure 4-6) and on the Arctic Islands.
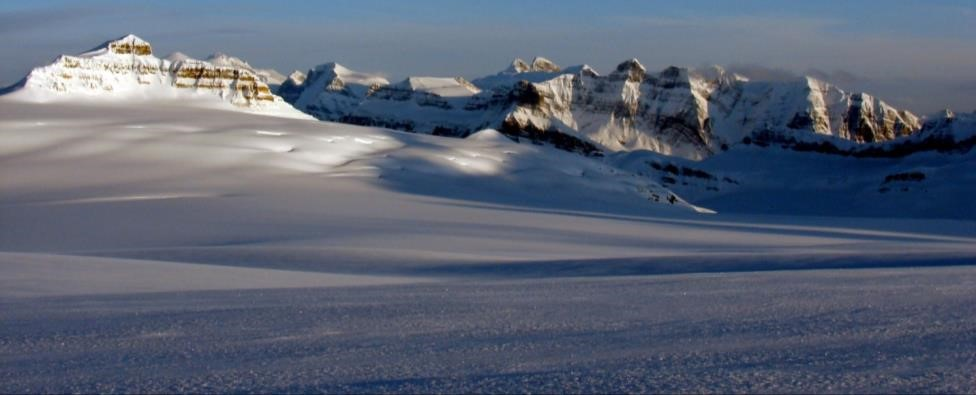
Hawthorne, R. (2011). View from camp atop Columbia Icefield looking south, Castleguard Mountain at left [Digital photo]. Wikimedia Commons. https://commons.wikimedia.org/wiki/File:Columbia_icefield_view.jpg
As illustrated in Figure 16.2.7, glaciers move because the surface of the ice is sloped and also because the force exerted by the overlying ice causes the lower ice to flow as a plastic solid. The rate of deformation is greatest towards the base of the glacier, but because the overlying ice always moves along with the lower ice, the upper part of the glacier always moves faster than the lower part. An alpine glacier flows downhill, but that’s only because the surface of the ice is sloped in that direction (Figure 16.2.4). A continental glacier flows from the area of thickest ice towards the continental margin where the ice is thinner. In both cases, ice accumulates in the higher-elevation part of the glacier because in those areas, not all of the winter snow melts in the subsequent summer (in other words, the glacier is always covered in snow), and the ice flows towards the area where mass is being lost due to melting (a.k.a. ablation) or to the collapse of the leading edge. The boundary between the zone of accumulation and the zone of ablation is the equilibrium line, which can be easily observed on a glacier in late summer as the boundary between areas still covered in snow, and those with exposed (and melting) ice (Figure 16.2.6). Above the equilibrium line, snow is constantly being converted to ice through the process described in Figure 16.2.5.
Glacial ice may or may not slide across the rocky surface that it rests upon. If the base is warmer than the melting point of ice (because of heat flow from the rock beneath), a film of water will form between the ice and its base, and sliding is likely. If the base is frozen, sliding is unlikely, and all of the movement will be caused by internal deformation. Warm-based glaciers tend to move faster than frozen-based glaciers. The temperature at the base of a glacier is dependent on the average annual temperature in the region and the thickness of the ice.
At all stages in the life of a glacier (except when it is very small, either because it is just starting to form or has nearly all melted away), the ice moves forward (down-slope in the case of an alpine glacier). Due to friction against the sides of the valley, an alpine glacier tends to move faster in the middle than at the sides (Figure 16.2.11). If the rate of production of new ice above the equilibrium line exceeds the rate of loss of ice below the equilibrium line, the glacier’s front end will advance. If those rates are the same, it will remain in the same place. If melting exceeds accumulation—as is the case with virtually all glaciers at present—the front end of the glacier will recede (Figure 4-7).
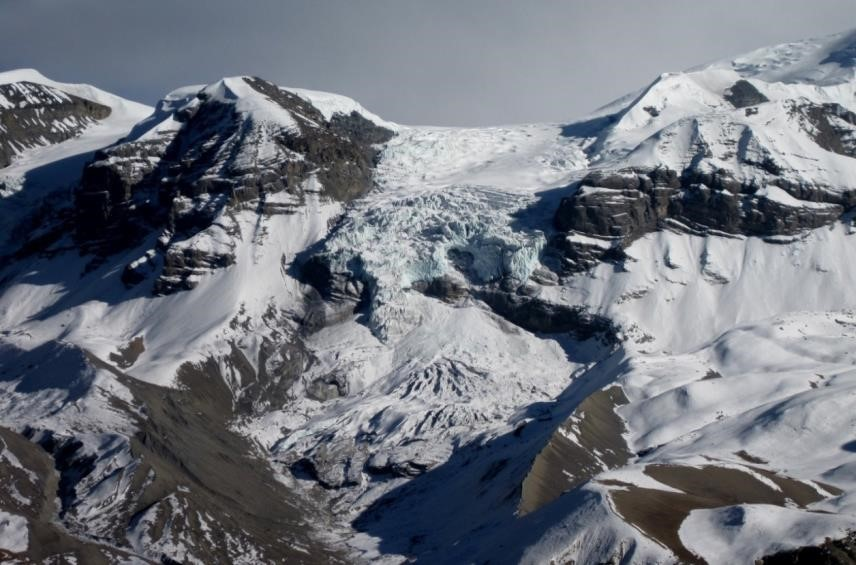
© Isaac Earle. Used with permission.
Completing Exercise 16.2 will help you to understand the processes of glacial motion and advance and retreat. Check your answer in the course textbook.
Section 16.3 summarizes the effects of glacial erosion, and as noted, continental glaciers produce substantially different erosional features than alpine glaciers. The key erosional landform of an alpine glacier is the U-shaped valley, with its steep sides and wide and relatively flat bottom, and the derivative features such as an arête that forms between two glaciers, a bowl-shaped cirque at the head of a U-shaped valley, a horn that forms between three or four cirques, and a hanging valley that is created where a smaller tributary joins a larger glacier that has eroded a deeper valley. Most of the important erosional features of alpine glaciation are illustrated from a variety of different perspectives in Figures 16.3.2, 16.3.3, 16.3.4, and 16.3.5 in the textbook, and also in Figure 4-8.
Take a close look at these figures and also complete Exercise 16.3 to help you ensure that you understand what alpine glacial erosion features look like.
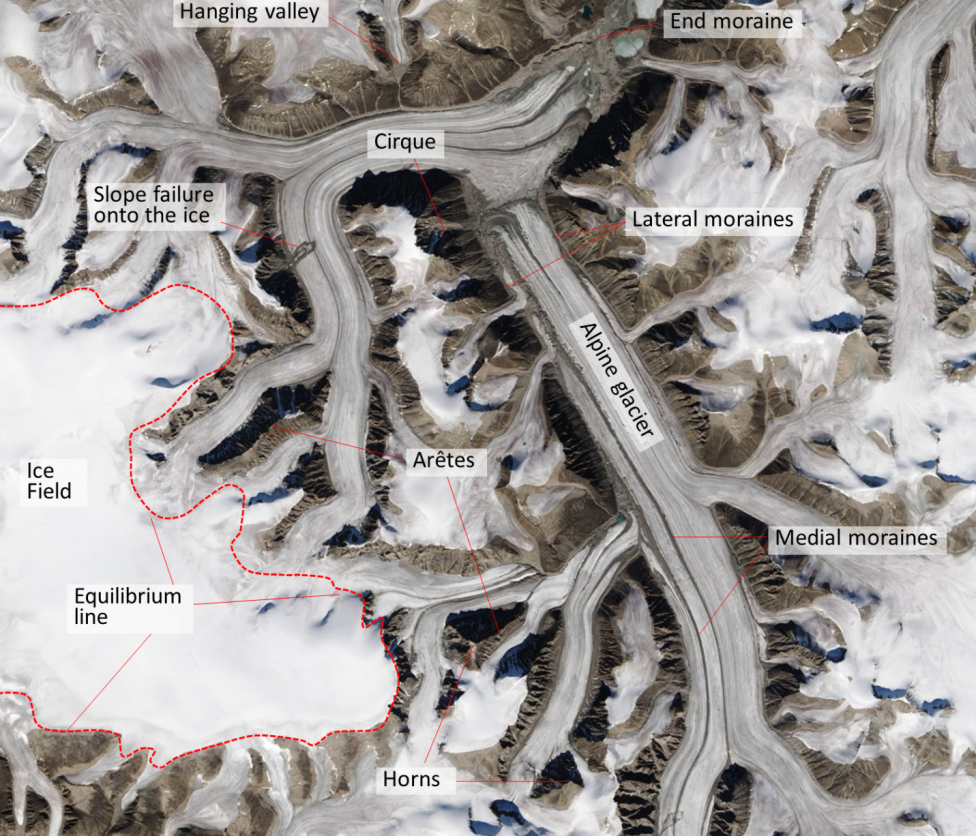
Adapted from Allen, J. (2015). Letter K: Sirmilik National Park [Digital image]. NASA Earth Observatory. http://earthobservatory.nasa.gov/IOTD/view.php?id=87216&src=ve.
The striking erosional features of alpine glaciation are not created beneath continental glaciers. Instead, continental glaciers create vast areas that have been eroded to a nearly flat plain, although streamlined features, such as drumlins (Figure 16.3.1) and roches moutonée, and smaller features such as glacial grooves and striations (Figure 16.3.8) are common.
As summarized in section 16.4, glaciers transport sediments in a wide variety of ways, including on top of the ice, within the ice, at the base of the ice, and, perhaps most important of all, in running water on top, within, and beneath the ice (Figure 16.4.2). Some of the deposits associated with glaciers are unique to glacial environments, the most obvious being till, which is pushed along beneath the ice as lodgement till, or which rides along within or on top of the ice and is deposited as ablation till when the ice melts (Figure 16.4.3). The important and unique characteristic of till is that it is unsorted and does not typically show any layering. Glaciofluvial sediments are fluvial (stream deposited) sediments that accumulate in a glacial environment. In many cases, they look very similar to normal fluvial deposits, and the only evidence of their glacial origin is their age and location. In some cases, direct evidence of glaciation exists, such as the presence of layers of till, or drop stones from ice floating on glacial lakes and streams (Figure 16.4.8a). Glaciolacustrine deposits are typically made up of fine sediments that accumulate in pro-glacial lakes, and typically have thin annual laminations related to summer and winter differences in meltwater production (Figure 16.4.8b).
Completing Exercise 16.4 will help you to understand where and how sediments are deposited in glacial regions. Some of the locations you are asked to identify may be underneath the ice.
Please answer the review questions at the end of Chapter 16.