Table of Contents
2-3 Metamorphic Rocks
Read Chapter 7 in Physical Geology.
Metamorphism and metamorphic rocks, which are described in Chapter 7 of your textbook, occupy the bottom part of the rock cycle, and represent the processes that occur between the lithification of sedimentary rocks and the partial melting that forms the magma for igneous rocks. The transition between lithification and metamorphism occurs where some of the original minerals of the sedimentary or igneous “parent” rock become unstable (typically owing to elevated temperatures), and new minerals start to form.
Section 7.1 summarizes the factors that control metamorphic processes. The mineral composition of the parent rock determines which elements are available to make new minerals (see Figure 7.1.1). The temperature and pressure determine which existing minerals will become unstable and which new ones will become stable during metamorphism. The existence of directed pressure affects the texture of the new rock (see Figure 7.1.1). Fluids (especially water) are important because they can facilitate the movement of ions within the crust, and also can promote the growth of new minerals. Finally, time is important because metamorphic processes are amongst the slowest of any geological processes.
Completing Exercise 7.1 will give you an understanding of the amount of time necessary for metamorphic processes.
As described in Section 7.2, the first criterion in the classification of metamorphic rocks is their texture. Some metamorphic rocks are foliated—meaning they have a planar fabric—and some are not foliated. Typically, the development of foliation depends on differences in the intensity of pressure in different directions during metamorphism, which normally is a product of plate convergence. Although some foliation forms simply because the rock is compressed more in one direction than in others (Figure 7.2.1), most foliation is a result of the preferential alignment of newly-forming minerals in a direction perpendicular to the main pressure direction (Figure 7.2.2). Planar minerals like mica and elongated minerals like amphibole are particularly important in imparting foliation to a rock because they grow with their long axes aligned.
An example of this process is shown in Figure 2-10, in which the crystals of mica have been forced to grow perpendicular to the main stress direction (pressure from the top and bottom in this view). Although the quartz and garnet formed under the same pressure conditions, these minerals are not elongated, and so they do not express the same degree of alignment.
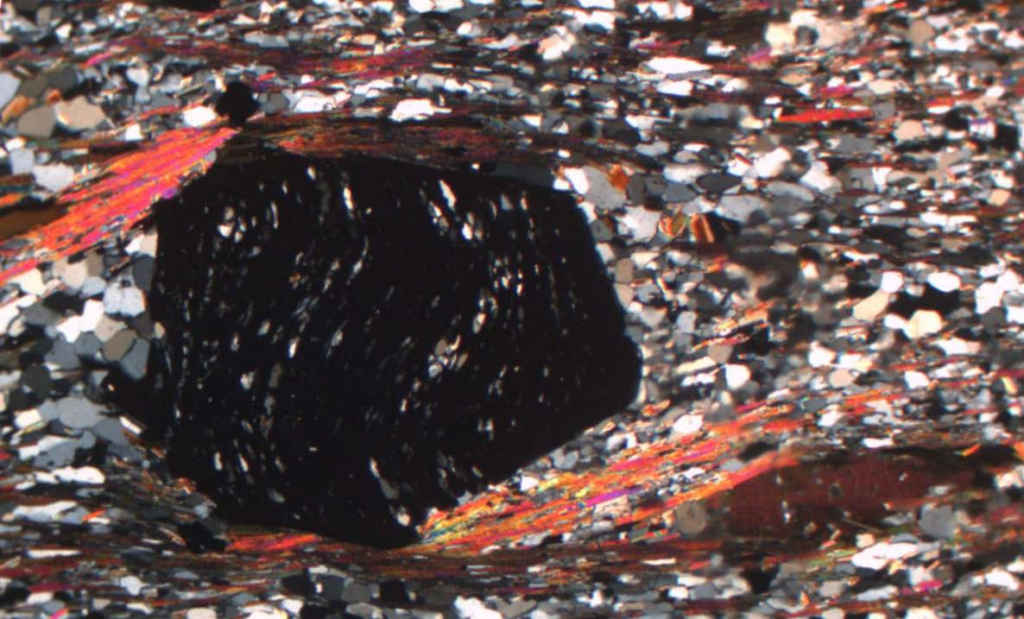
Dann, J. (2010). Thin section image of Garnet Mica Schist from Salangen, Norway (Digital image). Wikimedia Commons. https://commons.wikimedia.org/wiki/File:Thin_section_of_garnet-mica-schist.jpg
Section 7.2 describes the textures and mineralogy of the four main types of foliated metamorphic rock (slate, phyllite, schist, and gneiss). Make sure you are familiar with these.
Non-foliated metamorphic rocks are classified typically on the basis of their composition. They include marble (derived from limestone and consisting primarily of calcite), quartzite (derived from sandstone and consisting primarily of quartz), and hornfels (derived from mudstone or fine sandstone and consisting of a range of different minerals, including micas and quartz).
As shown in Figure 7.2.8, some rocks that appear to be non-foliated may actually be foliated at a microscopic scale. This is quite common in quartzite and marble, and is an important indicator of the conditions under which the metamorphism occurred.
Complete Exercise 7.2 to gain some practice in interpreting the characteristics of metamorphic rocks.
Section 7.3 includes a discussion of the relationships between plate tectonics and metamorphism. As summarized in Figure 7.3.1, sedimentary or igneous rocks that formed under one set of pressure and temperature conditions may be subjected to different conditions as a result of plate interactions. Under these different conditions, some of their minerals are no longer stable. For example, where continents collide and mountain ranges are formed (Figure 7.3.2), sedimentary rocks—which are comprised of minerals that formed under surface conditions (such as clays)—can be buried to depths of tens of kilometres and subjected to temperatures of several hundred degrees. Make sure you understand this environment of metamorphism, and also that which occurs near to sea-floor spreading ridges (Figure 7.3.3), and the contact metamorphism that occurs in the vicinity of an upper-crustal magma chamber (Figure 7.3.6).
Regional metamorphism, related to burial beneath mountain ranges and heating at spreading ridges, affects large volumes of rocks measured in thousands of cubic kilometres. In contrast, contact metamorphic zones (or aureoles) tend to be only metres to tens of metres in extent. As a result, the vast majority of metamorphic rock is formed under conditions of regional metamorphism, and most of it is foliated.
The geothermal gradient (the rate of increase in temperature with depth) differs in different geological environments. In a subduction zone, for example, the cold descending oceanic crust does not heat up as quickly with depth as does the continental crust in other areas. Conversely, in areas of volcanic activity, it’s possible to encounter hot rocks at much shallower depths than elsewhere. These differences in the geothermal gradient result in a wide range of pressure-temperature conditions within the crust, as is illustrated in Figure 7.3.7. Take a careful look at this diagram to assist you in visualizing where some of the different types of metamorphic rocks form, but don’t feel that you need to memorize these relationships. Completing Exercise 7.3 will help you to understand Figure 7.3.7.
Section 7.4 describes regional metamorphism in more detail and introduces the concept of metamorphic index minerals. Figure 7.4.1 shows some of the important index minerals in the context of temperature, but don’t forget that pressure also plays an important role in mineral stability, as we saw with respect to the minerals kyanite, andalusite, and sillimanite in Figure 7.1.1.
The processes commonly related to regional metamorphism—including continental collision and mountain building; thickening of the continental crust and isostatic adjustment of the crust-mantle boundary; development of metamorphic zonation related to burial depth; and finally, erosion, uplift, and isostatic readjustment—are illustrated in Figures 7.4.2 and 7.4.3, which are based on an example from Nova Scotia. Make sure that you understand how crustal thickening and differential crustal rebound following erosion play critical roles in determining the distribution of metamorphic zones in this situation. Exercise 7.4 may help you to understand these processes.
As described in Section 7.5, contact metamorphism occurs adjacent to igneous intrusions at relatively shallow depths in the crust. Differential pressure is not normally a major factor at shallow depths, so foliation is uncommon. Typical rock types formed include marble, quartzite, and hornfels. Complete Exercise 7.5 to reinforce your understanding of these rock types and processes.
Upper crustal intrusions have significant impacts on the distribution of heat near to the Earth’s surface, which can lead to the development of convection systems within groundwater. In addition, a great deal of water can be given off by a cooling body of magma, and when this water is dispersed into the surrounding rock, it can have significant effects on other surrounding rocks. These processes are illustrated in Figure 7.5.2 and described in the associated text. Several important types of ore deposits, including many of those found in British Columbia, can be formed under these conditions.
You are encouraged to answer the questions at the end of Chapter 7, and then check your answers in the textbook.